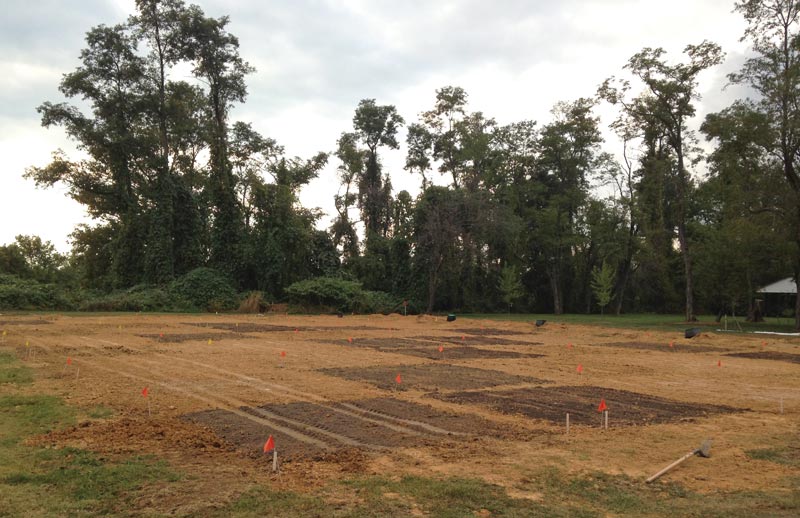
Figure 1. In August 2013, in preparation for this research project, vegetation and topsoil were removed and irrigation lines were installed at the study site, located at the Virginia Tech Turfgrass Research Center in Blacksburg, Va. Photo by Adam Boyd
Tall fescue (Festuca arundinacea Schreb.) is one of the most common grasses planted in the transition climate zone. Reductions in turfgrass quality can lead to soil erosion and diminished groundwater recharge and pollutant filtration (2). Inputs of nutrients and irrigation are often needed for establishment and maintenance of high-quality tall fescue turfgrass. Irrigating to replace 80% of reference evapotranspiration (ET) has been widely accepted for maintaining acceptable quality of a tall fescue turfgrass stand (3, 5). However, irrigation of large land areas in turfgrass can be costly and energy-intensive. Landowners and managers need to develop strategies that reduce irrigation inputs while maintaining turfgrass quality.
Land development exposes compacted subsoil after the removal or burial of vegetation and topsoil. Increased soil bulk density from soil compaction reduces water infiltration and water and nutrient uptake by altering the growth and distribution of roots (4, 6). In addition, removing or burying topsoil depletes surface soil organic matter and nutrients, especially nitrogen and phosphorus.
Biosolids, the nutrient-rich organic materials from the treatment of wastewater residuals, have had limited use in turfgrass fertilization. Wastewater treatment facilities have increasingly shifted toward improving the quality of biosolids so that they can be recycled into the soil. Biosolids that have undergone a process to further reduce pathogens (PFRP) and have also met the Code of Federal Regulations Title 40 (40 CFR) Part 503 pollutant concentration limits are termed “Class A” exceptional-quality biosolids (8).
Class A exceptional-quality biosolids have no application restrictions except for plant-available nitrogen and phosphorus. These improved-quality biosolids have more potential uses in urban turfgrass landscapes if they can be treated to improve handling and application. Reducing the water content (that is, dewatering) of the biosolids is one step in achieving improved handling and disposal. Adding materials that have a high ratio of carbon to nitrogen — for example, sawdust, mulch and paper products — can further reduce moisture content and improve aggregation, while the addition of sand can improve handling.
Biosolids normally have low nitrogen-to-phosphorus ratios. Therefore, repeated applications of biosolids at the agronomic nitrogen rate may result in the overapplication of phosphorus. Concerns about phosphorus loss to surface waters and more stringent regulations on phosphorus application make it even more important to develop turfgrass fertilization strategies for biosolids application that provide sufficient plant-available nitrogen and limit environmentally deleterious phosphorus.
Our objectives were to determine whether exceptional-quality biosolids of varying organic matter, nitrogen and phosphorus concentrations could be managed to improve tall fescue quality in a reduced-irrigation strategy; enhance properties of disturbed urban soil; provide sufficient plant-available nitrogen without increasing soil phosphorus to excessive concentrations; and improve turfgrass establishment, quality and growth.
Materials and methods
This study was conducted at the Virginia Tech Turfgrass Research Center in Blacksburg, Va., located in cold hardiness zone 6b. The experimental area was graded for an airport runway in the early 1940s but was never used. The area was converted to a tall fescue stand for several decades.
In August 2013, we killed off all vegetation and removed the topsoil that had accumulated, exposing the clayey subsoil. Subterranean irrigation pipes with pop-up heads were installed to irrigate eight equal areas of 68 feet Ă— 12 feet (20.7 meters Ă— 3.7 meters) (Figure 1, above). The soil was rototilled to 4 inches (10 centimeters) to level the study site for plot layout and incorporate fertilizer amendments on Sept. 11, 2013. The area was seeded with a tall fescue blend the next day. All remaining fertility applications were topdressed.
Experimental design
A split-plot randomized complete block design with four replications was used. The main plots were two summer irrigation treatments, and subplots were five fertility amendments. The irrigation treatments were: (1) no water applied during critical summer months unless necessary to keep vegetation alive; and (2) water applied to replenish 80% of ET during drought-prone summer months with high ET. The main plots were 68 feet Ă— 12 feet. Irrigation was withheld from all replicates of the non-irrigated main plot from May 31 to July 5, 2014, as conditions required irrigation to prevent crop failure. Rainy conditions minimized water stress and differences between main plot treatments in 2015. Therefore, irrigation was applied at 80% of ET for the remainder of the study period.
The five subplot treatments were synthetic fertilizer and four exceptional-quality biosolids byproducts applied annually to provide an estimated rate of 200 pounds plant-available nitrogen/acre (224 kilograms/hectare). Subplots were 12 feet Ă— 12 feet (3.66 meters Ă— 3.66 meters) and had 2-foot (0.61-meter) buffer strips between experimental units.
Soil benchmark sampling and analysis
Soil sampling and characterization were performed immediately following site preparation in August 2013. The soil test analysis showed pH of 5.1, 11 ppm Mehlich-1 phosphorus, 61 ppm Mehlich-1 potassium, 504 ppm Mehlich-1 calcium, 218 ppm Mehlich-1 magnesium, and 0.3% soil organic matter. Dolomitic limestone was applied uniformly to all treatments, with the goal of raising soil pH to 6.2. Particle-size analysis, measured via pipette method, determined the soil was in the textural class of clay (39.8% sand, 20.0% silt, 40.3% clay).
Fertility treatments and application
The five fertility treatments were four exceptional-quality biosolids byproducts and a synthetic fertilizer. Anaerobically digested, dewatered, Class A biosolids were obtained from an advanced wastewater treatment facility and biosolids generator (Alexandria Renew Enterprises, Alexandria, Va.). The dewatered biosolids product was used as a stand-alone treatment, and the biosolids product was blended with sand and sawdust for a second product at a 2:1:1 ratio (by dry mass), respectively.
The dewatered biosolids product (DBN) was applied at an annual rate of 200 pounds plant-available nitrogen/acre. The biosolids blended with sand and sawdust was applied at two rates. The first was applied at an annual rate of 200 pounds plant-available nitrogen/acre (BBN), and the second was applied at an annual phosphorus rate of 57 pounds/acre (6.38 grams/square meter) and supplemented with sulfur-coated urea fertilizer to total 200 pounds plant-available nitrogen/acre annually (BBP).
A composted biosolids was obtained from the Livingston composting facility in Spotsylvania County, Va. This material was produced by composting anaerobically digested, dewatered Class B biosolids with wood fines, creating an exceptional-quality biosolids compost applied at an annual rate of 200 pounds plant-available nitrogen/acre (CBN). The fifth fertility treatment was synthetic nitrogen fertilizer (sulfur-coated urea) applied at an annual rate of 200 pounds plant-available nitrogen/acre (FER). Samples of each organic amendment were collected prior to application and analyzed by A&L Eastern Laboratories (Richmond, Va.) (Table 1).
Application rates of biosolids byproducts were based on estimates of the organic nitrogen that would be mineralized during the first year after application. Virginia Department of Conservation and Recreation nitrogen mineralization rates are estimated to be 30% for anaerobically digested biosolids and 15% for composted biosolids. For the biosolids and sand and sawdust blends, the nitrogen mineralization rate of 20% was based on previous greenhouse studies (9). Application rates and dates are not reported here (but can be requested from the author).
Triple superphosphate (0-46-0) and muriate of potash (0-0-60) were applied to the synthetic fertilizer treatment plots and to the biosolids treatment plots as needed, based on the pre-treatment soil test results. Following tillage and incorporation of the fertility amendments in September 2013, the area was seeded with a tall fescue blend (32.75% Legitimate, 32.64% Chipper and 32.61% Aristotle) at a rate of 435 pounds/acre (487.57 kilograms/hectare) pure live seed.
Turfgrass analyses
Turfgrass clipping yield was collected every other week throughout the growing season by mowing a single pass of 1.6 feet Ă— 12 feet (0.48 meter Ă— 3.65 meters) down the center of each plot at a constant 3-inch (7.6-centimeter) height on a seven-day interval. Clippings were dried and the biomass recorded. The dried clipping biomass was ground and analyzed for leaf tissue nitrogen content. Clipping leaf tissue nitrogen accumulation was calculated as the product of biomass and nitrogen concentration.
Visual turfgrass assessment was determined every other week from spring to fall by visually integrating the turfgrass color, density, uniformity and leaf texture (7). Quality ratings were assigned by visual assessment on a scale of 1 to 9, where 9 = an ideal turfgrass stand, 6 = minimum acceptable quality, and 1 = dormant or dead turfgrass.
Normalized difference vegetation index (NDVI) spectral analysis, calculated as near-infrared (NIR) - red/[NIR + red] of each plot turfgrass canopy, was taken every other week throughout the growing season. A hand-held multispectral radiometer (Holland Scientific, Model ACS-430 Crop Circle), mounted to a Bag Boy Quad Plus pushcart (Bag Boy Co., Richmond, Va.) at a stationary height of 1.5 feet (0.45 meter) above the turf canopy, took an average of 100 readings per 12 feet (3.65 meters) of linear travel through the center of each plot to measure NDVI.
Soil chemical and physical measurements
Soil samples from September 2015 were analyzed for pH and Mehlich-1 extractable phosphorus, potassium, calcium, magnesium, zinc and manganese by the Virginia Tech Soil Testing Laboratory. At the conclusion of the study period (June 1, 2016), soil was sampled to a depth of 4 inches and measured for total soil organic carbon and nitrogen concentrations. Soil bulk density was determined by taking two cores per subplot on May 31, 2016, to a depth of 2 inches (5 centimeters) using method 3B6a by the Soil Survey Staff.
Results and discussion
Overall study statistical trends
Beneficial effects of soil organic carbon on turfgrass quality and persistence did not occur because of the short duration of the drought cycle imposed throughout the trial (five weeks in 2014). We decided to statistically analyze the results as an eight-replication randomized complete block design because of the lack of interaction between irrigation and amendment treatments on any variable.
Soil responses
Concentrations of Mehlich-1 phosphorus, calcium, magnesium, zinc and manganese in soil sampled in September 2015 were greater for CBN and BBN than for the other soil amendments (Table 2). The CBN and BBN treatments surpassed 55 ppm Mehlich-1 phosphorus, which would require biosolids to be applied at the phosphorus removal rate of tall fescue clippings in a growing season based on Virginia phosphorus management strategies (Table 2). Applying at the agronomic phosphorus rate for the BBP treatment resulted in a soil Mehlich-1 phosphorus concentration of 12 ppm (medium availability rating) in 2015. This phosphorus management strategy was protective of water quality, but it reduced turfgrass quality. Higher rates could likely be applied in the short term to improve turfgrass growth and quality without increasing the risk of water impairment.
The value of CBN and BBN can be seen throughout the trial period, as they had the greatest total soil nitrogen and organic carbon of all treatments in 2016 (Table 3). This was expected, because CBN and BBN had higher application rates in terms of total mass when compared with DBN and BBP. Soil bulk density of the FER and BBP treatments within the 0- to 2-inch depth were comparable at 70.5 pounds/cubic foot (1.13 grams/cubic centimeter) and 68.7 pounds/cubic foot (1.10 grams/cubic centimeter), respectively. The larger rates of organic carbon supplied by the DBN, BBN and CBN treatments reduced soil bulk density to 64.3, 60.6 and 52.4 pounds/cubic foot (1.03, 0.97 and 0.84 grams/cubic centimeter), respectively. Reduced soil bulk density would be expected to promote improved rooting conditions and a denser turfgrass stand, thus resulting in better turfgrass growth and quality with the highest carbon-amendment applications.
Turfgrass responses
The greatest biomass yield and leaf tissue nitrogen accumulation occurred with FER during the 2014 establishment year (Table 4). Lower-than-expected plant-available nitrogen from the biosolids-based products may have limited turfgrass establishment. Our plant-available nitrogen estimates were based on data from a greenhouse bioassay study using coarse-textured soil media (1). It is possible that in our soil system, the clayey soil provided increased adsorption sites for organic carbon and reduced nitrogen mineralization. After additional surface applications of fertility amendments in August 2014, increased biomass, leaf tissue nitrogen accumulation, NDVI and turfgrass quality occurred in biosolids-based treatments applied at the agronomic nitrogen rate (DBN, BBN and CBN) compared with the synthetic fertilizer (FER) treatment throughout the remainder of the study (Table 4). The continued mineralization of the original incorporated biosolids and surface applications in 2014 and early 2015 created a readily available pool of inorganic nitrogen to meet the tall fescue needs from June 2015 to June 2016 when no fertility amendments were applied.
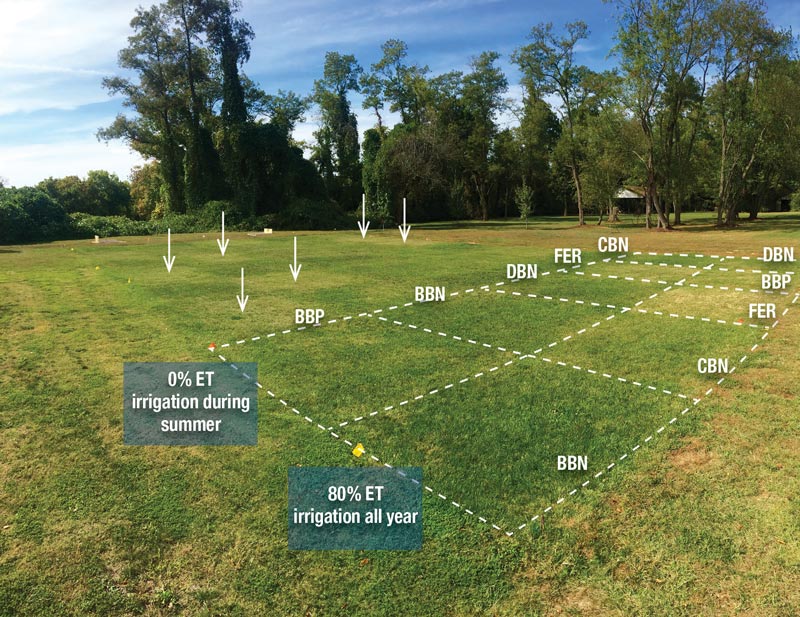
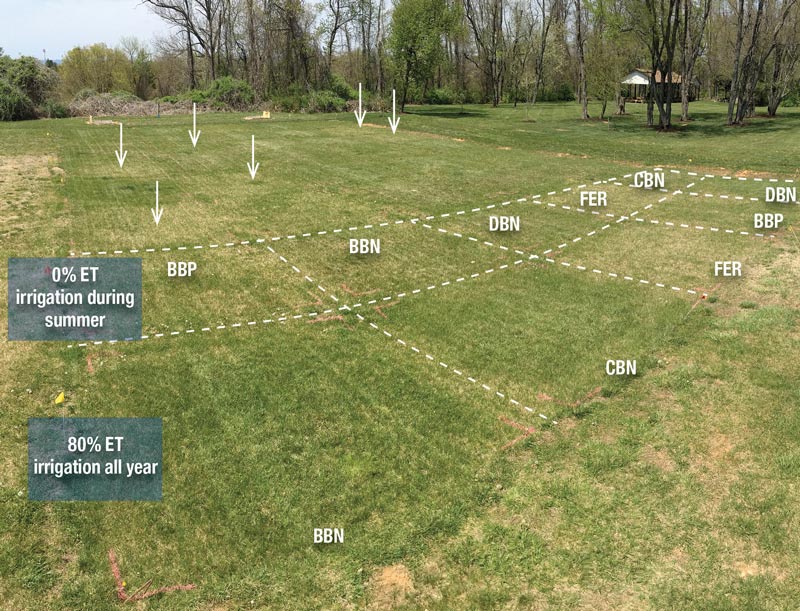
Figure 2. Top: This September 2015 photo of the research site shows the effects of fertility treatments with no summer irrigation and with 80% ET irrigation throughout the year. Bottom: The same study area on April 1, 2016. Fertility treatments were: FER, annually applied synthetic fertilizer nitrogen, phosphorus and potassium; DBN, biosolids applied annually at agronomic nitrogen rate; BBN, blended biosolids-sand-sawdust applied annually at agronomic nitrogen rate; BBP, blended biosolids-sand-sawdust applied annually at agronomic phosphorus rate plus supplemental fertilizer nitrogen; CBN, composted biosolids applied annually at agronomic nitrogen rate. Arrows indicate the additional replications of the FER treatment. Photos by Mike Badzmierowski
Turfgrass quality was greatest in the synthetic fertilizer (FER) treatment and maintained acceptable quality during 2014. The synthetic fertilizer treatment had only one measured date that was above minimum acceptable quality after 2014. Beginning in 2015, biosolids-based amendments applied at the agronomic nitrogen rate produced higher quality ratings for the remainder of the trial and maintained minimum acceptable quality from July 2014 through the remainder of the trial period, with the exception of the first sampling day of 2016 (a result of the turfgrass beginning to break winter dormancy) (Table 4). The biosolids-based products applied at the agronomic nitrogen rate were able to provide prolonged quality and improved quality on breaking winter dormancy (Figure 2, above).
The biosolids-sand-sawdust applied at the agronomic phosphorus rate supplemented with synthetic nitrogen (BBP) produced lower turfgrass quality than biosolids applied at the agronomic nitrogen rate. The presence of incompletely stable sawdust carbon may have resulted in nitrogen immobilization/lower nitrogen mineralization (despite the relatively low carbon-nitrogen ratio of 15:1). Reducing the amount of sawdust in this product could have led to a more desirable release of the synthetic fertilizer.
Conclusions
Biosolids-based products applied at the agronomic nitrogen rate provided a better long-term solution than synthetic fertilizer for the maintenance of tall fescue because the biosolids reduced soil bulk density and provided greater nitrogen availability, as indicated by leaf tissue nitrogen accumulation and the increased macro- and micro-nutrient concentrations delivered by the biosolids-based products. Additional research is needed on the effects of biosolids products on plant-available water and turfgrass persistence during drought.
Funding
The authors would like to thank DC Water and the Metropolitan Washington Council of Governments for funding of this research. We are appreciative of Alexandria Renew Enterprises and the Livingston composting facility for supplying the biosolids products. Funding for this work was provided in part by the Virginia Agricultural Experiment Station and the Hatch Program of the National Institute of Food and Agriculture, U.S. Department of Agriculture.
Acknowledgments
We thank our Virginia Tech colleagues Jonathan Dickerson, Julie Burger, Steve Nagle, Odiney Alvarez and Hunter Wyatt, without whose help this work could not have been completed.
The research says ...
- Wastewater treatment plants are improving the quality of biosolids produced for use as fertilizers, and some of them are now suitable for turfgrass use.
- One concern about biosolids is their low nitrogen-phosphorus ratio, which can result in excessive phosphorus applications if they are continually applied at the agronomic nitrogen rate over an extended period of time.
- Four biosolids products and a synthetic fertilizer were compared to determine whether they could be successfully used to grow tall fescue on degraded urban soil.
- The biosolids products were found to be more effective than synthetic fertilizer for improving turfgrass quality and growth and soil properties.
Literature cited
- Alvarez-Campos, O., G.K. Evanylo and M.J. Badzmierowski. 2018. Development and assessment of exceptional quality biosolids for urban gardens. Compost Science & Utilization 26(4):232-244.
- Beard, J.B., and R.L. Green. 1994. The role of turfgrasses in environmental protection and their benefits to humans. Journal of Environmental Quality 23:452-460. doi:10.2134/jeq1994.00472425002300030007x
- Carrow, R.N. 1995. Drought resistance aspects of turfgrasses in the southeast: Evapotranspiration and crop coefficients. Crop Science 35:1685-1690. doi:10.2135/cropsci1995.0011183X003500060029x
- Hamza, M.A., and W.K. Anderson. 2005. Soil compaction in cropping systems: A review of the nature, causes and possible solutions. Soil and Tillage Research 82:121-145. doi:https://doi.org/10.1016/j.still.2004.08.009
- Kopec, D.M., R.C. Shearman and T.P. Riordan. 1988. Evapotranspiration of tall fescue turf. HortScience 23:300-301.
- Kozlowski, T.T. 1999. Soil compaction and growth of woody plants. Scandinavian Journal
of Forest Research 14:596-619. doi:10.1080/02827589908540825
- Morris, K.N. 2000. Guidelines for using NTEP trial data. Golf Course Management 68(4):64-69.
- USEPA. 1994. A plain English guide to the EPA Part 503 Biosolids Rule. United States Environmental Protection Agency, Office of Wastewater Management, Washington, D.C.
- Yu, H.C., G.K. Evanylo and K. Haering. 2013. Comparisons of exceptional quality biosolids amendments as disturbed soil amendments. Poster 3010. https://scisoc.confex.com/crops/2013am/webprogram/Paper79328.html. ASA, CSSA and SSSA International Annual Meetings. Tampa, Fla. Nov. 3-6, 2013.
Mike J. Badzmierowski is a research associate, and Gregory K. Evanylo is a professor in the School of Plant and Environmental Sciences at Virginia Polytechnic and State University, Blacksburg, Va. Erik H. Ervin is chair and professor of turfgrass and horticultural systems at the University of Delaware, Newark, Del. Adam Boyd is a research associate in the Department of Crop, Soil and Environmental Sciences at Auburn University, Auburn, Ala. Carlyle Brewster is chair and professor of plant and environmental sciences at Clemson University, Clemson, S.C.